“We can trace out a boundary that predicts the loss of stability and the onset of fluctuations of a population.”
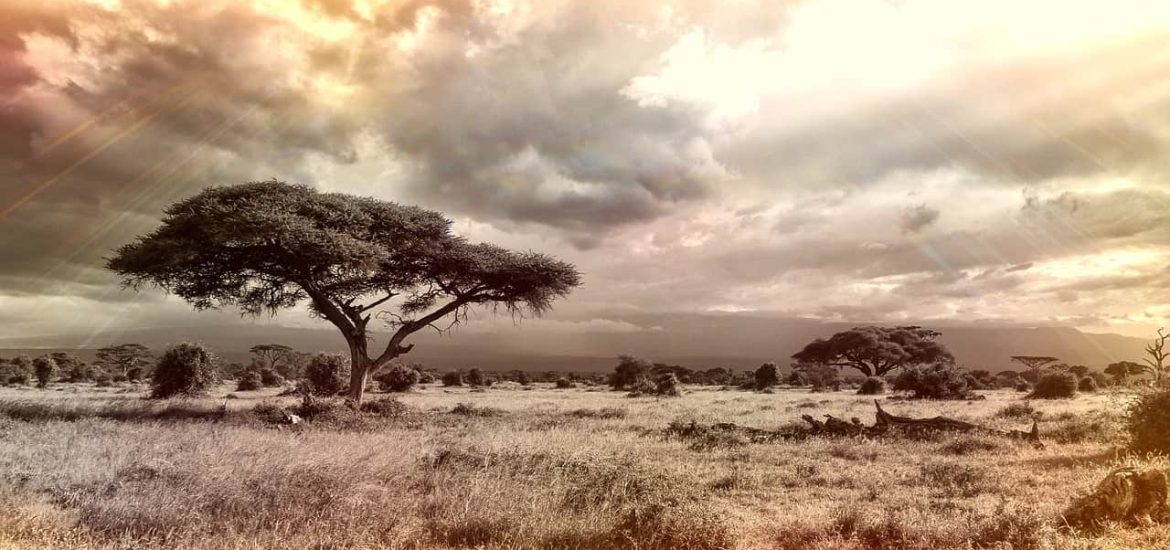
Scientists gain a better understanding of how ecosystems become unstable
Photo: Pixabay/cocoparisienne
Ecosystems are exceedingly complex systems wherein even relatively minor changes can trigger large-scale consequences. For all that, however, only knowing two variables can help us predict the health of an ecosystem. These, according to scientists at the Massachusetts Institute of Technology, are the number of species and the strength of their interactions.
The MIT researchers, who specialize in examining interspecies interactions among microbes during controlled experiments in the lab, set out to calculate what conditions were necessary for communities to move from one state to another during three distinct states of development.
This approach yielded a “phase diagram,” which resembles diagrams employed by physicists to track the conditions required for water to transition from solid to liquid to gas form.
“What’s amazing and wonderful about a phase diagram is that it summarizes a great deal of information in a very simple form. We can trace out a boundary that predicts the loss of stability and the onset of fluctuations of a population,” explains Jeff Gore, a professor of physics at MIT who led the project, the findings of which have just been published in a study.
“In order to see phase transitions in the lab, it really is necessary to have experimental communities where you can turn the knobs yourself and make quantitative measurements of what’s happening,” he adds.
Gore and his colleagues have previously demonstrated how competitive and cooperative behaviors affect populations, which helped them identify early-warning signs of population collapse in larger ecosystems. In the process, Gore decided to test some of the predictions that theoretical physicists have made regarding the dynamics of large, complex ecosystems.
“One of those predictions was that ecosystems move through phases of varying stability based on the number of species in the community and the degree of interaction between species. Under this framework, the type of interaction — predatory, competitive, or cooperative — doesn’t matter. Only the strength of the interaction matters,” the scientists explain.
To test that prediction, Gore and his colleagues created communities of bacteria of up to 48 species. In each the researchers controlled the number of species by forming different communities with different sets of species.
They then strengthened the interactions between species by increasing the amount of food available, which allowed populations to become larger while causing environmental changes such as increased acidification. The tests confirmed the prediction that at first each community would exist in a phase of “stable full existence,” wherein all species coexist with little or no interference.
However, when the number of species or their interactions increased, the communities entered a second phase called “stable partial coexistence,” in which populations remain stable while some species became extinct. “The overall community remained in a stable state, meaning that the population returns to a state of equilibrium after some species go extinct,” the scientists explain.
Once the number of species or the strength of their interactions increased even further, the communities entered a third phase, which was marked by increased instability with far more dramatic fluctuations in population. Even as some species went extinct, however, these ecosystems still often had a larger overall number of species that survived.
“Using this data, [we] were able to draw a phase diagram that describes how ecosystems change based on just two factors: number of species and strength of interactions between them,” the scientists note.
“This is analogous to how physicists are able to describe changes in the behavior of water based on only two conditions: temperature and pressure. Detailed knowledge of the exact speed and position of each molecule of water is not needed,” they add.
This insight into ecosystems is important because accurate predictions can now be made about them based on just a few known facts.
“While we cannot access all biological mechanisms and parameters in a complex ecosystem, we demonstrate that its diversity and dynamics may be emergent phenomena that can be predicted from just a few aggregate properties of the ecological community: species pool size and statistics of interspecies interactions,” says Jiliang Hu, a graduate student at MIT who was a key part of the project.
A phase diagram such as this, Gore adds, can help ecologists make predictions about what might be happening in natural ecosystems such as forests even with little information available because all they will need to know is the number of resident species and how closely they interact.
“We can make predictions or statements about what the community is going to do, even in the absence of detailed knowledge of what’s going on,” Gore says. “We don’t even know which species are helping or hurting which other species. These predictions are based purely on the statistical distribution of the interactions within this complex community.”
Further work at MIT is now examining the flow of new species between otherwise isolated populations such as those similar to island ecosystems affects the dynamics of those populations. “This could help to shed light on how islands are able to maintain species diversity even when extinctions occur,” they note.